Translate this page into:
An observational study of auditory-evoked potential mismatch negativity response in individuals at high genetic risk for schizophrenia
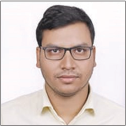
*Corresponding author: Rakesh Kumar Jangde, Department of Psychiatry, Chhattisgarh Institute of Medical Sciences, Bilaspur, Chhattisgarh, India. rakeshcims10@gmail.com
-
Received: ,
Accepted: ,
How to cite this article: Jangde RK, Umesh S, Goyal N, Khess C. An observational study of auditory-evoked potential mismatch negativity response in individuals at high genetic risk for schizophrenia. Arch Biol Psychiatry. 2024;2:25-9. doi: 10.25259/ABP_6_2024
Abstract
Objectives:
This study presents a novel approach by investigating the abnormal mismatch negativity (MMN) found during response to auditory-evoked potential (ERP) in schizophrenia (SZ) patients. Our unique focus is on the genetic high-risk (GHR) for SZ, comparing it with healthy controls (HC). In addition, we aim to illuminate the interrelationship of MMN with socio-occupational functioning in the GHR group, a novel aspect of our research.
Material and Methods:
We conducted this study with a rigorous methodology, ensuring the reliability and validity of our findings. Thirty first-degree relatives of SZ patients (GHR) who met the inclusion and exclusion criteria were recruited by purposive sampling and compared with thirty HCs. We collected sociodemographic and clinical data from all study participants, which were assessed by the Family Interview of Genetic Studies. GHR was evaluated for current socio-occupation functioning using the Social and Occupational Functioning Assessment Scale (SOFAS). All the participants underwent 128-channel electroencephalographic recording using an auditory-evoked responses paradigm. The MMN was calculated at the frontal electrode clusters, ensuring accurate and reliable data collection.
Results:
The study revealed a statistically significant difference in frontal MMN latency between GHR and HC. Moreover, a positive relationship was noted between the SOFAS score and MMN amplitude, and a negative relationship was found between the latencies at the frontal region.
Conclusion:
Our research has made a significant breakthrough in understanding SZ. Our findings reveal that individuals with a genetic susceptibility to SZ exhibit a unique response in a particular test known as MMN. This test measures the automatic auditory change detection mechanism, which detects minor alterations in the acoustic environment and is referred to as MMN. This newfound insight could prove instrumental in developing more effective diagnoses and treatments for SZ.
Keywords
Genetic high risk
Mismatch negativity
Socio-occupational functioning
Schizophrenia
INTRODUCTION
Schizophrenia (SZ) has heritable quantitative biological measures related to disease risk.[1] Numerous studies have identified intermediate phenotypes, aiding in understanding disease mechanisms and developing better treatments. The ideal intermediate phenotype for brain disease is to measure a heritable and state-independent form, which is co-segregated from the illness in families and expressed at a higher rate than the healthy population.[2] Intermediate phenotype studies in SZ research have identified several important neurocognitive, neurophysiological, and anatomical illness markers. Abnormal event-related potentials (ERPs) such as P50 and P300 have been found in first-degree relatives (FDRs) of SZ patients.[3] Changes in evoked gamma electroencephalography (EEG) have been associated with several critical cognitive functions. Evoked gamma band response to auditory tones is poorly synchronized in chronic SZ patients,[4] first episode, patients, and unaffected relatives.[5] Patients at clinically high risk for psychosis demonstrated significantly decreased evoked power in the gamma band relative to healthy controls (HCs). However, the magnitude of the abnormality in gamma-band evoked power was less pronounced in clinical high-risk psychosis.[6] The findings of reduced evoked power gamma band responses (GBRs) in unaffected co-twins of patients with SZ suggest that reduced auditory-evoked gamma power may reflect the risk of developing SZ.[7]
Small changes in the acoustic environment engage an automatic auditory change detection mechanism, reflected in the mismatch negativity (MMN). Repeated or familiar deviant events evoke a response recorded with EEG. Occasional variations of acoustic stimuli in a regular sequence of sounds generate an event-related potential (ERP) in the brain known as MMN. Subtracting the ERP wave from infrequent deviant sounds from the ERP to frequent regular or standard sounds yields a difference in waveform between 100 and 240 ms.[8] Temporal and frontal areas of topographic scalp maps exhibit the most vigorous intensity of MMN.[9] the MMN might be associated with preattentive cognitive operations in audition ten due to its automatic nature. People with SZ have exhibited deficits in MMN production.[10,11] Earlier, a study found reduced MMN amplitude in chronic SZ patients but not in their unaffected relatives.[12] However, various studies have shown that MMN impairment is more significant among high-risk samples that later convert to SZ.[6,13] MMN deficits also represent a core neurophysiological dysfunction that is linked to global impairments in everyday functioning in SZ patients.[14] Based on this, we intended to understand the patterns of MMN deficits in the genetic high-risk (GHR) group and their interrelationship with their current socio-occupational functioning.
MATERIAL AND METHODS
Patient characteristics
This was a hospital-based and cross-sectional study conducted in a tertiary referral center in India in the year between January 2017 and January 2019. The tertiary referral center for psychiatric patients has a wide catchment area, including Jharkhand, Bihar, West Bengal, North Eastern states, Uttar Pradesh, Madhya Pradesh, and neighboring countries such as Nepal and Bangladesh. Participants included 30 FDRs of SZ (GHR), 30 age- and sex-matched HCs from the same geographic location who provided consent and had no family history of mental illness were included in the study. Participants were informed of the study, and written consent was obtained. Subjects were right-handed males aged more than 18 years. FDRs of patients diagnosed with SZ meeting the diagnostic criteria as per the International Classification of Diseases, Tenth Revision-Diagnostic Criteria for Research were included in the study. The Family Interview for Genetic Studies was conducted to gather diagnostic information about the FDRs and HC being studied. Participants were excluded if there was a history of epilepsy, chronic physical illness, organic brain disorder, or major psychiatric illness. HC was recruited from the vicinity of the institute and scored <3 on general health questionnaire-12.[15] The study was approved by the Central Institute of Psychiatry, Ranchi Ethics Committee, and it conformed to the provisions of the Declaration of Helsinki.
EEG recording and analyses
The recording was conducted in a sound-attenuated room using a 128-channel EEG system (EBNeuro®, Italy) using a custom-made cap using a 10–10 system montage arrangement.[16] We used system-integrated headphones to provide pure tones of two distinct frequencies, 1000 Hz (standard tone) and 3000 Hz (deviant tone). They were calibrated to 60 dB sound pressure level, lasting for 300 ms with a varying intertrial interval of 2000–5000 ms. Two hundred standard tones and 50 deviant tones were provided using the oddball paradigm. The EEG recording was sampled at 256 Hz, and electrooculography (EOG) was used to identify ocular movements and eyeblink. The data were exported to European data format (EDF)+ format for preprocessing. Two researchers (US and NG) visually inspected the data independently, and artifacts were manually recognized and rejected. Independent component analysis was performed on the raw data, and the first two components (IC1 and IC2) correlated with the EOG signals. Their topographies, which were typical of eye movements, were rejected. The EEG was resampled to 512 Hz, filtered (0–35 Hz; 50 Hz AC), and the baseline was corrected. One second, 50 epochs (deviant) and 200 epochs (standard) were extracted for further analysis. Max negative peaks between 100 and 240 ms from the averaged deviant and standard tone were calculated. MMN was calculated by subtracting the negative peak of standard tones from the deviant tones. A grand average file was finally generated group-wise for visual representation [Figure 1a]. The data were processed using MATLAB R2018a and BRAINSTORM.[17]
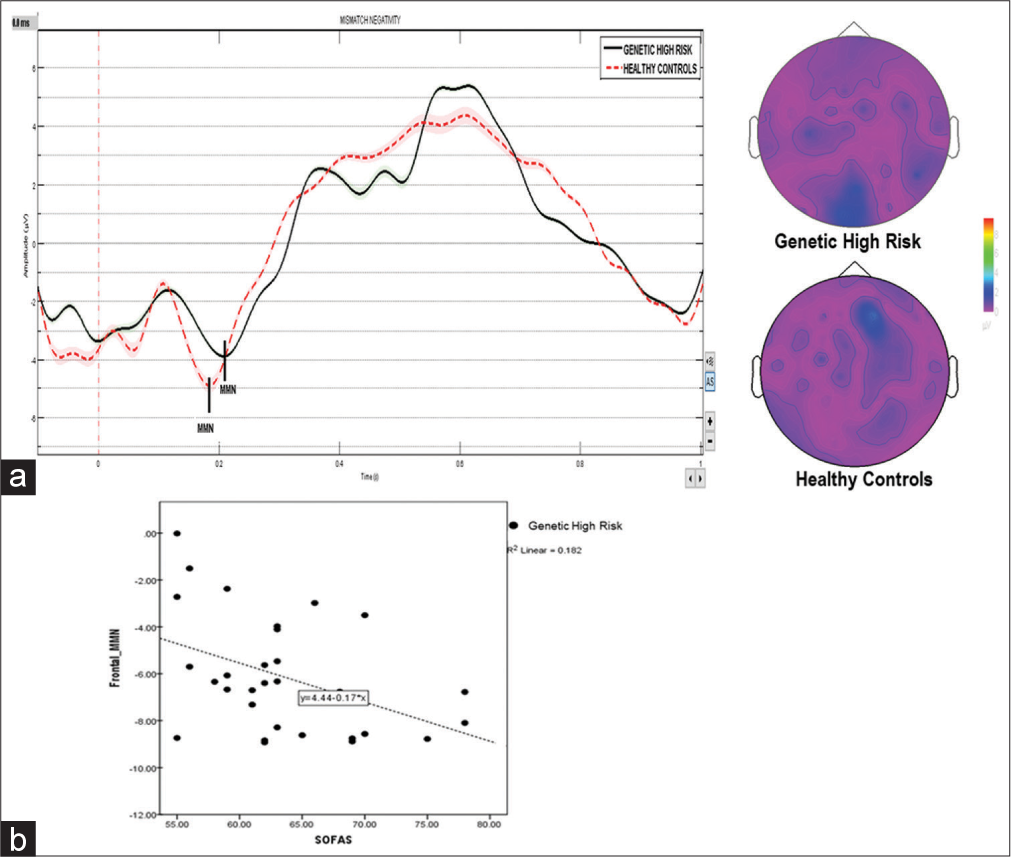
- (a) Reduced mismatch negativity (MMN) amplitude and increased latency in the genetic high-risk group compared to healthy controls. The color bar displays contrast of topoplot. (b) A scatterplot displaying a negative correlation between frontal MMN and the current social and occupational functioning assessment scale score (SOFAS).
RESULTS
Genetic High Risk (GHR) and HC groups were compared across various sociodemographic characteristics [Table 1]. There was no significant difference between the two groups regarding religion, education, occupation, socioeconomic status, family income, and habitat. The mean age of the GHR group was 29.63 ± 6.59 years, and 30.00 ± 5.02 years for the HC group. The mean frontal MMN amplitude in the GHR group was −4.44 ± 1.94 and −5.76 ± 3.25 in the HC group (t = −1.89, P = 0.06). Mean frontal MMN latency in the GHR group was 198.89 ± 29.87 ms and 182.98 ± 31.97 ms in the HC group (t = −1.99, P = 0.051). Pearson correlation coefficients between the current Social and Occupational Functioning Assessment Scale (SOFAS) score and MMN showed a negative correlation (r = −0.426, P = 0.02) to amplitude and a negative correlation to latency (r = −381, P = 0.04). The scatterplot shows the relationship between the two variables [Figure 1b].
Variable | GHR (n=30) Mean±SD/n(%) | HC (n=30) Mean±SD/n(%) | t/χ2 | P |
---|---|---|---|---|
Age (in years) | 29.63±6.59 | 30.00±5.02 | −0.242 | 0.809 |
Education (in degree) | ||||
Secondary/higher secondary | 14 (46.7) | 14 (46.7) | 0.000 | 1.000 |
Graduate/above | 16 (53.3) | 16 (53.3) | ||
Socioeconomic status | ||||
Low | 16 (53.3) | 17 (56.7) | 1.138f | 0.553 |
Middle | 14 (46.7) | 12 (40.0) | ||
High | 0 (0.0) | 1 (3.3) | ||
Habitat | ||||
Rural | 12 (40.0) | 14 (46.7) | 0.271 | 0.602 |
Urban | 18 (60) | 16 (53.3) | ||
Family income (in INR ) | ||||
0–20000 | 18 (60) | 17 (56.7) | 0.119 | 0.942 |
20000–50000 | 7 (23.3) | 7 (23.3) | ||
>50000 | 5 (16.7) | 6 (20) | ||
Religion | ||||
Hindu | 25 (83.3) | 28 (93.3) | 1.456 | 0.228 |
Muslim | 5 (16.7) | 2 (16.7) | ||
Occupation | ||||
Employed | 17 (56.7) | 19 (63.3) | 1.112f | 0.561 |
Unemployed | 1 (3.3) | 0 (0.0) | ||
Other | 12 (40) | 11 (36.7) |
GHR: Genetic high risk, HC: Healthy controls, SD: Standard deviation, f: Comparison of sociodemographic profile of Genetic High Risk and Healthy Controls, INR: Indian Rupees.
DISCUSSION
Numerous studies have been conducted to identify markers of vulnerability to SZ. These studies have found that the automatic auditory change detection mechanism, reflected in the MMN, which occurs in response to small changes in the acoustic environment, is significantly impaired in patients with SZ.[13] Deficits in MMN frequency associated with illness duration are suggestive that MMN may index ongoing neuropathological changes in the auditory cortex in SZ.[18] At the neurochemical and anatomical levels, MMN has been shown to reflect impaired N-methyl-D-aspartate receptor function at the level of the supratemporal auditory cortex in SZ.[19,20] The extent of MMN reduction strongly predicts global functioning and degree of independent living and social functions,[14,21,22] linguistic ability,[23] and cognition.[24,25]
MMN deficits in unaffected FDRs would prove that it is genetically linked rather than affected by the disease process.[26] The researchers reported reduced MMN amplitude in non-psychotic FDRs of patients with SZ. Another study revealed that MMN impairment likely reflected a vulnerability to disease progression in clinical high-risk populations rather than a genetic risk for the condition.[13] In SZ, it is largely unrelated to the duration of illness after the first few years of disease, indicating that the impairment is not progressive throughout the life span. MMN impairment is a consequence of higher-order auditory expectancy deficits in SZ patients and those who are at elevated clinical risk for converting to psychosis.
In our study, we investigated MMN in HCs and FDRs with GHR. In this study, there was a significant difference in frontal MMN latency in HCs and FDRs of SZ patients. These findings were similar to studies that were conducted earlier. In the study,[27] the latency of MMN was found to be significantly reduced in GHR subjects converting to first-episode psychosis compared with non-converters and may contribute not only to the prediction of conversion but also to more individualized risk estimation and, thus, risk-adapted prevention. At risk mental state, subjects who later converted to overt SZ elicited reduced duration of MMN amplitudes in frontal and central leads compared with non-converters and normal subjects.[12,28]
In this study, we also conducted a correlational analysis of frontal MMN with GHR (Risk Mental state) with SOFAS, which shows that as the SOFAS score decreases, the MMN amplitude and latency decrease. These findings were similar to a study in the past in which SZ patients exhibited reduced MMN activity at frontocentral electrode sites compared to HCs. Within the SZ sample, more significant MMN activity at frontocentral sites correlated with better work and independent living.[21] Earlier studies have also shown a correlation between smaller MMN amplitudes and poor socio-occupational functioning in a SZ sample.[11]
MMN amplitude was reduced in SZ patients and correlated with their impaired day-to-day function level. Only in patients, bilateral gray matter reduction in Heschl’s gyrus, as well as motor and executive regions of the frontal cortex, correlated with reduced MMN amplitude in response to frequency deviants, while the reduced gray matter in the right Heschl’s gyrus also associated with reduced MMN to duration deviants. These findings further support the importance of MMN reduction in SZ by linking frontotemporal cerebral gray matter pathology to an automatically generated event-related potential index of daily functioning.[8] In conclusion, this study showed a trending difference in MMN latency and amplitude in individuals with high genetic risk compared to HCs. Some limitations included the lack of comprehensive assessment of at-risk groups and psychopathological measures of schizotypy. Future studies may focus on following individuals who are GHR and predict their psychosis conversion.
Limitations
The study has several limitations that should be acknowledged. First, the sample is overrepresented in North India, which may limit the generalizability of the findings to participants from other parts of the country. This study includes only male FDRs of patients with SZ. Thus, gender-specific results could not be generalizable for the whole schizophrenic population. A more diverse sample encompassing regions such as the West and South of India would have enhanced the study’s comprehensiveness.
CONCLUSION
We concluded that our findings reveal that individuals with a genetic susceptibility to SZ exhibit a unique response in a particular test known as MMN. This test measures the automatic auditory change detection mechanism, which detects minor alterations in the acoustic environment and is referred to as MMN. This newfound insight could prove instrumental in developing more effective diagnoses and treatments for SZ.
Authors’ contributions
1. RJ-Data collection and manuscript preparation and editing. 2. US study design, data analysis, and manuscript editing, 3. NG-Data supervision and study design, 4. CRJ-Study design and supervision.
Ethical approval
The research/study is approved by Ethics Committee, Central Institute of Psychiatry, Ranchi, number 899, dated 3rd May 2024.
Declaration of patient consent
The authors certify that they have obtained all appropriate patient consent.
Conflicts of interest
There are no conflicts of interest.
Use of artificial intelligence (AI)-assisted technology for manuscript preparation
The authors confirm that there was no use of artificial intelligence (AI)-assisted technology for assisting in the writing or editing of the manuscript, and no images were manipulated using AI.
Financial support and sponsorship
Nil.
References
- Genetics of schizophrenia and the new millennium: Progress and pitfalls. Am J Hum Genet. 2001;68:299-312.
- [CrossRef] [PubMed] [Google Scholar]
- The endophenotype concept in psychiatry: Etymology and strategic intentions. Am J Psychiatry. 2003;160:636-45.
- [CrossRef] [PubMed] [Google Scholar]
- Meta-analysis of the P300 and P50 waveforms in schizophrenia. Schizophr Res. 2004;70:315-29.
- [CrossRef] [PubMed] [Google Scholar]
- Event-related EEG time-frequency analysis: An overview of measures and an analysis of early gamma band phase locking in schizophrenia. Schizophr Bull. 2008;34:907-26.
- [CrossRef] [PubMed] [Google Scholar]
- Reduced auditory evoked gamma band response and cognitive processing deficits in first episode schizophrenia. World J Biol Psychiatry. 2015;16:387-97.
- [CrossRef] [PubMed] [Google Scholar]
- Automatic auditory processing deficits in schizophrenia and clinical high-risk patients: Forecasting psychosis risk with mismatch negativity. Biol Psychiatry. 2014;75:459-69.
- [CrossRef] [PubMed] [Google Scholar]
- The early auditory gamma-band response is heritable and a putative endophenotype of schizophrenia. Schizophr Bull. 2011;37:778-87.
- [CrossRef] [PubMed] [Google Scholar]
- Gray matter deficits, mismatch negativity, and outcomes in schizophrenia. Schizophr Bull. 2011;37:131-40.
- [CrossRef] [PubMed] [Google Scholar]
- Auditory frequency discrimination and event-related potentials. Electroencephalogr Clin Neurophysiol. 1985;62:437-48.
- [CrossRef] [PubMed] [Google Scholar]
- The perception of speech sounds by the human brain as reflected by the mismatch negativity (MMN) and its magnetic equivalent (MMNm) Psychophysiology. 2001;38:1-21.
- [CrossRef] [PubMed] [Google Scholar]
- Neural substrates of normal and impaired preattentive sensory discrimination in large cohorts of nonpsychiatric subjects and schizophrenia patients as indexed by MMN and P3a change detection responses. Neuroimage. 2013;66:594-603.
- [CrossRef] [PubMed] [Google Scholar]
- Reduced mismatch negativity predates the onset of psychosis. Schizophr Res. 2012;134:42-8.
- [CrossRef] [PubMed] [Google Scholar]
- A meta-analysis of mismatch negativity in schizophrenia: From clinical risk to disease specificity and progression. Biol Psychiatry. 2016;79:980-7.
- [CrossRef] [PubMed] [Google Scholar]
- Mismatch negativity deficits are associated with poor functioning in schizophrenia patients. Arch Gen Psychiatry. 2005;62:127-36.
- [CrossRef] [PubMed] [Google Scholar]
- A scaled version of the General Health Questionnaire. Psychol Med. 1979;9:139-45.
- [CrossRef] [PubMed] [Google Scholar]
- 10/20, 10/10, and 10/5 systems revisited: Their validity as relative head-surface-based positioning systems. Neuroimage. 2007;34:1600-11.
- [CrossRef] [PubMed] [Google Scholar]
- Brainstorm: A user-friendly application for MEG/EEG analysis. Comput Intell Neurosci. 2011;2011:879716.
- [CrossRef] [PubMed] [Google Scholar]
- Mismatch negativity in schizophrenia: A meta-analysis. Schizophr Res. 2005;76:1-23.
- [CrossRef] [PubMed] [Google Scholar]
- NMDA receptor and schizophrenia: A brief history. Schizophr Bull. 2012;38:920-6.
- [CrossRef] [PubMed] [Google Scholar]
- Glutamatergic modulation of auditory information processing in the human brain. Biol Psychiatry. 2012;71:969-77.
- [CrossRef] [PubMed] [Google Scholar]
- Mismatch negativity, social cognition, and functioning in schizophrenia patients. Biol Psychiatry. 2010;67:940-7.
- [CrossRef] [PubMed] [Google Scholar]
- Automatic sensory information processing abnormalities across the illness course of schizophrenia. Psychol Med. 2012;6:1-5.
- [CrossRef] [PubMed] [Google Scholar]
- Profile of auditory information-processing deficits in schizophrenia. Psychiatry Res. 2009;165:27-37.
- [CrossRef] [PubMed] [Google Scholar]
- Mismatch negativity potentials and cognitive impairment in schizophrenia. Schizophr Res. 2004;69:203-17.
- [CrossRef] [PubMed] [Google Scholar]
- Cortical substrates and functional correlates of auditory deviance processing deficits in schizophrenia. Neuroimage Clin. 2014;6:424-37.
- [CrossRef] [PubMed] [Google Scholar]
- Amplitude reduction of the mismatch negativity in first-degree relatives of patients with schizophrenia. Neurosci Lett. 2001;309:185-8.
- [CrossRef] [PubMed] [Google Scholar]
- Prediction of psychosis by mismatch negativity. Biol Psychiatry. 2011;69:959-66.
- [CrossRef] [PubMed] [Google Scholar]
- Mismatch negativity and cognitive performance for the prediction of psychosis in subjects with at-risk mental state. PLoS One. 2013;8:e54080.
- [CrossRef] [PubMed] [Google Scholar]